Article: Optical Spectrum Analysis: Understanding Light’s Frequency Domain
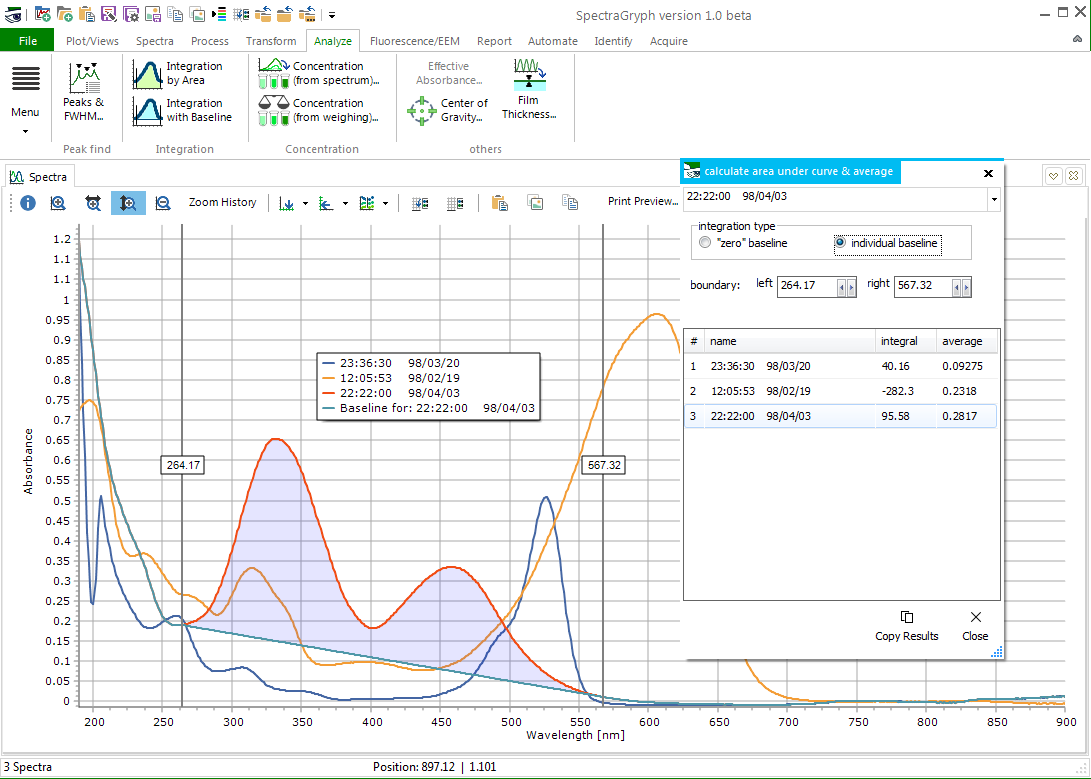
Optical Spectrum Analysis: Understanding Light’s Frequency Domain
Optical spectrum analysis is the process of examining the distribution of light power across different wavelengths or colors. In essence, it’s like taking a beam of light and breaking it down into a “rainbow” to see what wavelengths are present and how strong each is. This concept of spectrum analysis is not unique to optics – engineers also perform spectrum analysis in radio (RF) and audio domains, measuring signal power versus frequency. An optical spectrum simply refers to this frequency-domain view for light: it shows how the energy of a light source or signal is spread over wavelength
Light dispersion through a prism: a continuum of colors reveals the optical spectrum of the source. Optical spectrum analyzers (OSAs) use similar dispersion principles to separate light by wavelength.
In everyday terms, you can witness an optical spectrum when sunlight passes through a prism or water droplets to create a rainbow – the white light is separated into its constituent colors (wavelengths). An Optical Spectrum Analyzer (OSA) is an instrument designed to do this quantification and display of light’s spectrum in a precise, controlled way. It measures and plots optical power (on the vertical axis) versus wavelength or optical frequency (on the horizontal axis)
How Optical Spectrum Analyzers Work
To analyze an optical spectrum, the OSA must separate incoming light into its component wavelengths and measure each component’s power. In practice, most OSAs achieve this using dispersive elements or filters, combined with sensitive photodetectors. The incoming light is typically fed into the OSA either through a fiber-optic input (connecting a fiber cable to the device) or a free-space aperture where a collimated beam enters the instrument
Diffraction Gratings and Prisms:
A common method to spatially separate wavelengths is by using a diffraction grating (a finely ruled mirror or transmission grating) or, in some cases, a prism. As the light hits the grating or prism, different wavelengths bend at different angles due to diffraction or refraction, spreading out the spectrum much like the prism example above. OSAs often employ a diffraction grating inside a monochromator assembly. In a classic grating-based OSA, the grating is rotated to direct different wavelengths sequentially onto a narrow output slit and detector
Tunable Filter Sweeping:
An alternative design uses tunable optical filters, such as a scanning Fabry–Pérot interferometer. In this method, incoming light enters a resonant cavity formed by two parallel mirrors. By adjusting the mirror spacing (using a piezoelectric element), the cavity’s resonant wavelength is swept through the spectrum
Detection and Power Measurement:
No matter how the wavelengths are separated, the OSA ultimately measures optical power at each wavelength using a photodetector (typically a photodiode). The photodiode converts the light into an electrical current proportional to the optical power
Free-Space vs. Fiber-Coupled Inputs:
Some optical spectrum analyzers are optimized for fiber-optic inputs – you connect a fiber carrying the light, and an internal collimator directs the beam to the dispersive element. This is ideal for fiber communications signals. Other OSAs (especially those used in photonics research) support free-space input: you can shine a laser beam directly into an aperture or through a lens. For example, Thorlabs’ line of OSAs allows both FC fiber input and free-space beam input, accommodating a variety of sources
Comparisons with Electrical Spectrum Analyzers:
While OSAs and electrical spectrum analyzers share the basic concept of displaying power vs. frequency, there are key differences. Electrical/RF spectrum analyzers typically downconvert high frequency signals to an intermediate frequency and use electronic filters (or FFT algorithms) to sweep across frequencies. In contrast, an OSA must optically disperse or filter the actual light. There’s no way to “mix down” an optical frequency to baseband without losing the optical characteristics (unless using advanced coherent detection, which essentially turns it into an RF signal – a specialized case). As a result, OSAs deal directly with optical wavelengths, and the span of interest (commonly 800–1700 nm for telecom and near-IR devices, or other bands for different instruments) is constrained by detector sensitivity and grating coatings.
Applications of Optical Spectrum Analyzers
Optical spectrum analyzers are versatile instruments with applications spanning telecommunications, photonics research, laser engineering, sensing, and education. By providing detailed insight into the spectral content of light, OSAs enable users to diagnose issues and characterize sources far beyond what a simple power meter or light source could indicate. Below are some common and advanced use cases:
Example of a portable optical spectrum analyzer in field use: the Viavi JDSU T-BERD 2000 mainframe with a COSA-4055 CWDM OSA module. Such handheld OSAs allow engineers to analyze fiber-optic channel spectra and signal quality on-site, combining portability with lab-grade measurement capabilities.
- Telecommunications (WDM Networks): OSAs are crucial in fiber-optic communications, especially in WDM (Wavelength Division Multiplexing) networks. They resolve each channel in DWDM and CWDM systems, measuring power, center wavelength, and ensuring proper spacing (e.g., 100 GHz or 50 GHz in DWDM). Telecom engineers rely on OSAs to verify wavelengths and monitor optical signal-to-noise ratio (OSNR), which becomes critical after multiple EDFA amplifier spans. High-performance OSAs help calculate OSNR by displaying channel peaks and noise levels.
- Photonics Research (Broadband Sources and Components): In optical R&D, analyzing a source or device's spectrum is essential. OSAs characterize supercontinuum generators, broadband LEDs/SLDs, frequency combs, and other wideband sources. For instance, a supercontinuum laser emits light from visible to infrared in one beam—only an OSA can map its full spectral range and intensity. Researchers use OSAs to measure mode-locked laser pulses, optical spectra in nonlinear experiments, and wavelength-selective components like fiber Bragg gratings. By sweeping a tunable laser or using a broadband source, an OSA plots transmission or reflection spectra, acting as a spectrophotometer. Unlike fixed filters or power meters, OSAs provide a complete spectral view. Labs often use multiple OSAs for different ranges, as no single detector covers 400 nm to 2000+ nm with equal sensitivity. Instruments like the Thorlabs OSA20x series even extend into the mid-infrared (5–12 µm) for chemical sensing. Whether analyzing ultra-broadband sources or fine spectral ripples, OSAs deliver critical data for deeper insights.
- Laser Tuning and Diagnostics: Anyone working with lasers must understand their spectral properties, and OSAs are essential for laser tuning, linewidth measurement, and side-mode analysis. When adjusting a tunable laser, an OSA verifies the output wavelength and power. It helps engineers distinguish between single-frequency and multimode lasers by displaying longitudinal modes and quantifying side-mode suppression ratio (SMSR). For mode-locked lasers or frequency combs, OSAs provide a quick view of the comb envelope, though they can't resolve individual comb lines. In laser manufacturing, OSAs ensure lasers meet wavelength specs, aid in wavelength calibration, and diagnose issues like unexpected secondary lines or amplified spontaneous emission. Acting as the "eyes" of laser engineers, OSAs reveal critical spectral details beyond simple power readings.
- Optical Sensing and Metrology: Optical spectrum analyzers find uses beyond communications – for instance, in sensing applications and precision metrology. Many chemical sensors and environmental monitors work on optical absorption or emission spectra (think of how a sodium lamp has distinct yellow lines, or how gas absorption can produce narrow dips at specific wavelengths). An OSA can be used as a small-scale spectrometer to detect these signatures. For example, in gas sensing, one might shine a broadband source through a sample and use an OSA to detect absorption lines that indicate the presence of certain gases. OSAs have been used to identify gas absorption lines in spectroscopy setups.
- Education and Laboratory Use: OSAs are valuable tools in educational labs for optics and photonics, allowing students to observe spectra firsthand. Instead of just learning that an LED has a wider spectrum than a laser, students can measure each with an OSA and see the difference in real time. OSAs help demonstrate concepts like spectral bandwidth, filtering, and diffraction—for example, measuring the spectrum of a white light source and observing changes when optical filters are applied. They also enable exploration of emission lines from gas discharge lamps, providing calibrated wavelength readings. In university research labs, OSAs support a wide range of experiments, helping verify or analyze wavelength content. They are as essential to an optics lab as oscilloscopes are to electronics labs. Educational use also teaches students how to interpret OSA readouts, understand terms like dBm (logarithmic power unit), and recognize artifacts such as noise or etalon effects. Through hands-on experience, students gain familiarity with spectrum analysis as an integral part of working with optical systems.
Advantages of Spectrum Analysis vs. Basic Optical Tools
Why invest in an optical spectrum analyzer when one already has simpler instruments like optical power meters and stable light sources? The answer lies in the depth of information provided by spectral analysis. A basic optical power meter, for instance, only tells you the total optical power (or power in a broad wavelength range if a filter is used). It cannot tell how that power is distributed in wavelength. If you have multiple lasers or wavelength channels combined, a power meter will just sum them up, possibly leaving you blind to the presence of individual signals. An OSA, on the other hand, will separately show each wavelength’s power. This means if one channel drops or a spurious wavelength appears, the OSA makes it immediately obvious, whereas a power meter might barely register a change in the total.
Consider a scenario in telecom: you measure optical power on a fiber and it seems fine, but data isn’t going through correctly. A power meter might show, say, 0 dBm, implying “good” power. Hook up an OSA and you might discover that all that power is actually coming from an ASE (Amplified Spontaneous Emission) noise source and the actual data-carrying laser line is missing! This kind of diagnostic is only possible with spectral analysis. Similarly, when aligning a tunable laser, having an OSA is invaluable – without it, you could be lasing at the wrong wavelength and not know until the system fails to respond. A laser wavelength meter gives a number readout of wavelength, but an OSA actually shows the entire spectral content, which is more informative if there are sidebands or multiple lines. In fiber optic link troubleshooting, a common simple tool is a visual fault locator (a red laser pointer to find breaks) or a broadband light source and power meter to check loss. These tell you nothing about wavelength-dependent issues. In contrast, an OSA can identify problems like a filter passing the wrong band or an attenuator that is wavelength-selective.
Another advantage of OSAs is their ability to measure in-band optical noise and spectral shape. For optical amplifiers (like EDFAs), simply measuring gain with a power meter at one wavelength doesn’t reveal the amplifier’s ASE noise profile. With an OSA, you can measure the amplified signal as well as the out-of-band ASE levels and thus compute noise figures or OSNR. This is crucial in system design and compliance testing. Moreover, OSAs often have built-in analysis functions – for example, many can automatically find and label peaks (wavelength and power of each channel), compute OSNR by interpolation, measure spectral width (FWHM), and even perform pass/fail tests against a template (useful in manufacturing to check if a laser’s spectrum falls within specs). These are things a simple light source or power meter setup cannot do.
It should be noted that for absolute power accuracy, a well-calibrated optical power meter is typically more accurate than an OSA. Spectrum analyzers (optical or RF) trade a bit of absolute accuracy for frequency resolution. For instance, an OSA might have ±0.5 dB absolute accuracy on power, whereas a good power meter might be ±0.1 dB. If you only need total power, the power meter is the right tool. But the moment you need any spectral information – which is often the case in complex optical systems – the OSA becomes indispensable despite a slight compromise in precision. In practice, engineers use them in complement: the power meter for quick single-wavelength power checks and an OSA for detailed spectral scans. Thankfully, many modern OSAs are calibrated well enough that they double as decent power meters on any one wavelength (many have a “zero-span” mode to measure power at a fixed wavelength over time, effectively acting as a narrowband power meter).
In summary, optical spectrum analysis provides a complete picture of the optical signal that simpler instruments cannot offer. It uncovers the what and where of the light in terms of wavelength content. This is vital for debugging issues, optimizing system performance, and ensuring devices meet their specifications. Whether you’re deploying a DWDM telecom network, developing a new laser, or studying the emission of a novel optical source, an OSA empowers you with the detailed spectral insight needed to proceed with confidence.
Conclusion
Optical spectrum analyzers are a cornerstone of modern optical engineering and research. By bridging the gap between the time-domain world of fiber optics and the frequency-domain view of optical spectra, OSAs enable us to see light in all its complexity. We’ve discussed how they work – using diffraction gratings, prisms, or interferometric filters to dissect light – and how they differ from their electrical counterparts. We’ve explored a range of applications from cutting-edge telecom networks ensuring every channel is on target, to laser labs fine-tuning beams, to sensing applications detecting subtle spectral signatures. Throughout all these scenarios, the OSA proves to be an incredibly powerful diagnostic and analytical tool. It provides not just a measurement, but a visual story of the light’s composition.
For professionals and enthusiasts working with optical systems, understanding and leveraging optical spectrum analysis is crucial. The good news is that accessing this technology has never been easier. There are compact, field-ready OSAs for on-site troubleshooting, high-resolution bench units for laboratory precision, and even PC-connected spectrometer modules for specific wavelength ranges.
TT Instruments offers a range of Optical Spectrum Analyzers from leading manufacturers like EXFO and Viavi – from handheld modules to high-end lab instruments – to suit various needs (see our OSA product collection for examples). While this article has focused on the principles and uses, getting hands-on with an OSA is the best way to appreciate what spectrum analysis can reveal. In an era where optics and photonics are increasingly central (from 5G fronthaul fiber links to quantum communications and LIDAR systems), mastering tools like the OSA cements one’s technical credibility and opens the door to deeper insights.
The author is a Technical Lead at TT Instruments, a company specializing in top-quality test, fiber optic, and electronic test equipment. With an ISO 9001:2015 certification, TT Instruments guarantees reliable, high-performance products, along with huge discounts and express worldwide delivery, making it a trusted provider in the telecom, fiber optic, and electronic testing sectors.
References & Further Reading:
- EXFO White Paper – “Why OSAs are a better choice for spectral analysis than optical channel monitors” (EXFO Inc., 2018) – Detailed comparison of OSAs vs. embedded optical monitors.
- Viavi Solutions Application Note – “What is an Optical Spectrum Analyzer?” – Covers OSA principles and key parameters like wavelength accuracy and OSNR.
- Yokogawa Technical Note – “Measuring the OSNR of Modulated DWDM Signals Using an OSA” – Discusses techniques for accurate OSNR measurement in modern coherent systems.
- Thorlabs Engineering Workshop – “Optical Spectrum Analyzers” – Demonstrates different OSA models (including mid-IR FT-OSA) and their use cases in telecom and spectroscopy
- RP Photonics Encyclopedia – “Optical Spectrum” and “Optical Spectrum Analyzers” – Provides fundamental definitions and overviews of various OSA operating principles
- Keysight (Agilent) Application Note 5963-7145E – “Fundamentals of Optical Spectrum Analysis” – An older but comprehensive guide to OSA design, including diffraction grating monochromators and Fabry-Perot etalons